CCE: fisiologia
Autores: Guy Rebillard, Rémy Pujol
Ayuda a la realización: Nuno Trigueiros-Cunha
A característica fisiológica mais importante das CCEs é a electromotricidade: elas respondem à transdução mecanoelétrica ( de forma semelhante às CCI) por um mecanismo de de transdução inversa (eletromecânica). É um mecanismo ativo de amplificação que dá à cóclea excecionais propriedades de sensibilidade e selectividade em frequência.
O amplificador coclear
Ao longo dos anos 80, a compreensão da fisiologia coclear defrontou-se com uma contradição: por um lado, os neurofisiologistas descreviam uma excecional selectividade em frequência das fibras do nervo auditivo, e por outro, a teoria da onda viajante de Békésy que concebia a cóclea como um filtro passivo incapaz de discriminar essa seletividade frequencial. No entanto, desde 1948 um físico, Gold, propunha um “processo mecânico ativo” que poderia ser responsável por essa seletividade!
Dois resultados experimentais vieram comprometer a conceção da “cóclea passiva”: a descoberta das otoemissões acústicas e a medição precisa da vibração do órgão espiral (membrana basilar) em resposta a um som puro que mostrava que a concordância em frequência se fazia antes da mensagem auditiva ser detetada no nervo auditivo.
O duplo mecanismo de transdução
Como para as CCI, as vibrações acústicas provocam um movimento dos cílios das CCE que desencadeia uma modulação do potencial destas células. Assim, no caso dum som puro, os cílios vão oscilar de forma sinusoidal o que vai induzir uma sucessão de despolarizações e repolarizações das CCE.Esta atividade elétrica pode ser registada experimentalmente e designa-se potencial microfónico. Contrariamente ao que se passa nas CCI, as variações de potencial provocam alterações da forma das CCE. Quando estas células despolarizam elas encolhem-se e que quando repolarizam alongam-se. A alteração do comprimento das CCE é devida à modificação da forma duma proteína muito abundante na sua membrana celular, a prestina, sob o efeito das mudanças de potencial. As CCE desempenham um papel de osciladores que amplificam localmente a vibração da membrana basilar. Esta propriedade das CCE aumenta a sensibilidade da cóclea em cerca de 50dB e melhora a sua capacidade de codificar de forma diferente frequências muito próximas. Assim sendo, o papel das CCE é primordial na função auditiva.
Para um som puro, o mecanismo ativo amplifica (cerca de 50dB) a vibração da membrana basilar (o que aumenta a sensibilidade coclear) numa porção muito estreita do órgão espiral (de Corti). Duas frequências muito próximas podem assim ativar zonas distintas da cóclea, permitindo a distinção uma da outra (seletividade em frequência).Esta discriminação frequêncial (tuning) depende diretamente das propriedades eletromotrizes das CCE e é reproduzida a nível das fibras do nervo auditivo, ao qual é fielmente transmitida pelas CCI.
Eletromotricidade
A eletromotricidade foi descoberta em 1983, em CCE dissociadas. Posteriormente, esta propriedade das CCE foi profundamente estudada. Trata-se dum mecanismo rápido (podendo atingir frequências muito elevadas de até 20kHz, pelo menos), que não depende nem do cálcio nem do ATP; não consumindo diretamente energia (apesar da fisiologia geral da CCE ter, obviamente, necessidade de energia ATP dependente).
A contração das CCE é o resultado do somatório das contrações de muitos “motores” elementares localizados na parede lateral da CCE. Hoje pensa-se que a despolarização da CCE (entrada de K+ no momento da estimulação sonora) modifica a configuração duma proteína transmembranar, a PRESTINA, que desempenha o papel de “motor elementar” (ver animação abaixo). Esta teoria foi recentemente confirmada com a clonagem do gene da prestina e a sua deleção, anulando a propriedade eletromotriz destas células.
S. Blatrix de G. Rebillard e R. Pujol
A despolarização das CCE desloca aniões, provavelmente Cl-, dos seus locais de ligação à prestina para o citoplasma.
Este deslocamento de cargas elétricas induz o encurtamento da proteína que se traduz no encurtamento da CCE.
Inversamente, quando a CCE se repolariza, os aniões ligam-se novamente às prestina levando ao seu alongamento, o que conduz ao alongamento da célula.
Nota: Paralelamente com a eletromotricidade, as CCE isoladas revelaram outras capacidades contrateis: por exemplo, a contração lenta cálcio dependente, que modula a eletromotricidade e que é desencadeada “in vivo” pelo sistema eferente medial.
Hoje, ainda não se sabe qual o papel fisiológico desta modulação eferente das CCE: Será um sistema de proteção? ou um sistema da atenuação do mecanismo ativo implicado no “efeito cocktail”.
Membrana lateral e complexo submembranoso duma CCE
As propriedades eletromotrizes das CCE está diretamente relacionada com a estrutura singular da sua membrana lateral (porção entre a placa cuticular e nível do núcleo) e dum complexo de organelos submembranosos.
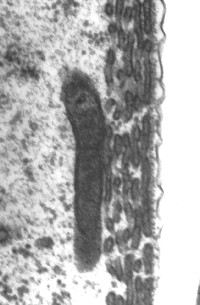
Cisternas de retículo endoplasmático alinham-se entre a membrana plasmática e as mitocôndrias. O número destas estruturas varia da base (1 só camada de cisternas: imagem da direita) para o ápice (4-5: imagem da esquerda).
Entre a primeira camada de cisternas e a membrana plasmática são visíveis pilares e outras microestruturas que formam o complexo citoesqueleto submembranoso (ver esquema abaixo).
Imagens MET : R. Pujol e M. Lenoir / Escala: 100 nm
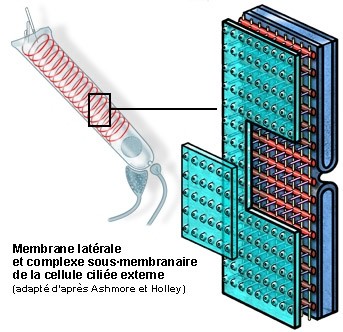
S. Blatrix, de Ashmore e Holley
Esquema da organização da membrana plasmática lateral e do citoesqueleto submembranoso.
Os três componentes do complexo submembranoso são claramente visíveis entre a membrana (verde, parcialmente levantada) e a primeira camada de cisternas do reticulo endoplasmático (azul):
- os filamentos de actina (vermelho) que envolvem as CCE, provavelmente com a forma dum mola helicoidal (ver esquema da célula inteira) com um passo de cerca de 100nm;
- os filamentos de espectina (ou fimbrina, em amarelo) arranjam-se longitudinalmente e espaçada de cerca de 50 nm;
- os pilares (violeta) dispõem-se perpendicularmente à membrana
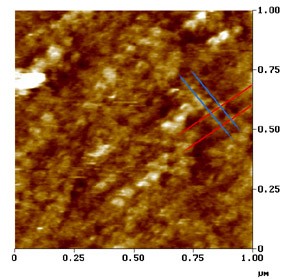
Le Grimellec, Lenoir, Pujol et al.
Imagem de microscopia de força atómica.
Superfície externa da membrana lateral duma CCE.
Neste µm² de membrana observa-se o arranjo particular das proteínas da membrana: os traços oblíquos indicam a direção em que as proteínas se encontram alinhadas, a intervalos de aprox. 50 nm (traços azuis) e de 100 nm (traços vermelhos) : ver esquema.
Estas proteínas, que parecem representar a porção transmembranosa dos pilares e que poderiam ser os ¨motores¨ das CCE, apresentam forma cónica e, por vezes, um poro central visível.